Mini Review
Creative Commons, CC-BY
Heme Enzymes: Nature’s Versatile Catalysts
*Corresponding author: Manjistha Mukherjee, Department of Chemistry, University of Basel 24a Mattenstrasse, Basel, CH-4058, Switzerland.
Received:June 01, 2022; Published:June 09, 2022
DOI: 10.34297/AJBSR.2022.16.002244
Abstract
Heme enzymes are ubiquitous in nature. Although these enzymes contain the same Fe-protoporphyrin IX cofactor in their active site, they exhibit different structural and functional activities. This brief review focuses on the reasons for this diversity and mentions some attempts to mimic the structural and functional activity of these heme enzymes. It also briefly discusses efforts to expand their catalytic repertoire to enable new chemical transformations.
Introduction
Naturally occurring metallo-enzymes are nature’s own catalysts [1]. The heme superfamily of enzymes is one of these well-known metallo-enzymes that exhibit versatile activity [2]. This group of enzymes contains a heme group that is either covalently or noncovalently attached to the active site. The versatile activities of these enzymes include intermolecular electron transfer (cytochrome c; Cyt c) [3] and selective reduction of O2 to H2O (cytochrome c oxidase; CcO, Figure 1C) [4] during mitochondrial respiration, which are well documented. It also transports and stores O2, e.g., hemoglobin (Hb) and myoglobin (Mb, Figure 1A). In addition, the heme is also involved in the activation small molecules to achieve useful chemical transformations. From heteroatom incorporation (oxygenase incorporates oxygen atoms into the substrates and chloroperoxidase; CPO: involved in halogenation of the substrates) [5] to oxidation (Heme peroxidase) [2], these heme enzymes play a crucial and central role in xenobiotic degradation and hormone synthesis. This brief review is focused on the reasons for the diverse reactivity in both biological enzymatic systems and their abiotic mimics. It also summarizes the use of the active site of these heme enzymes to engineer artificial metallo-enzymes for novel chemical transformations.
Reason for the Diverse Functional Activity
The differential reactivity of heme enzymes containing essentially the same heme (Fe bound to protoporphyrin IX) cofactor has been attributed to differences in their proximal ligand and distal superstructure [6,7]. Years of research have examined the effects of these two factors to explain the differences in the functional activity of these enzymes. The structural difference in the proximal ligand binding of these heme enzymes is very striking and clearly affects the electronic structure of the enzymes in their resting state [8]. That is why, the resting electronic state of Hb, Mb, CcO, and peroxidase, which contain histidine as an axial ligand, is very different compared with cytochrome P450 (Cyt P450; monooxygenase: incorporates an oxygen atom into inert C-H bonds; Figure 1B) and CPO, which have cysteine as an axial ligand. On the contrary, the variations in the distal superstructures (e.g., hydrogen bonds, local electrostatics, proton transfer channels, etc.) may or may not significantly affect the electronic structure of the metal centre in the resting. However, it has been proven beyond doubt that the difference between the proximal ligand and the distal residues drastically affects the reactivity of these active sites by tuning the transition states of different possible reaction pathways [9]. The different proximal ligands are thought to have different “push effects” by exerting different electron density on the central metal [5]. On the other hand, the different distal environments of some of these active enzyme sites are thought to exert different “pull” effects that stabilize the intermediates during the catalytic cycle [5]. The study of the individual contributions of the different proximal ligands and distal environments to the various reactivities of these proteins has proven to be quite difficult. The main problem in studying these effects in an active protein site by single point mutations of the active site residues is the possible change in protein conformation associated with them. This is where the synthetic mimics of these enzymes come into the rescue (Figure 1).
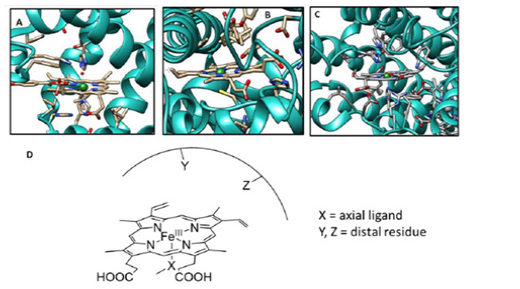
Figure 1: Active site structure of few heme based metallo-enzymes A. Myoglobin (pdb id: 2V1J), B. Cytochrome P450 (pdb id: 1AKD), and C. Cytochrome c Oxidase (pdb id: 3X2Q. D. Schematic representation of heme enzyme active site demonstrating the effect of axial ligand (X) and distal secondary residue (Y, Z).
Synthetic Models of Heme Enzymes
To date, several synthetic mimics of heme enzymes have been presented. The iron “pickle fence” porphyrin synthesized by Collman and co-workers is an excellent functional mimic of Mb [10]. The Fe-Cu active site model of CcO synthesized by the same group demonstrated the role of the distal metal binding site and the effects of electron transfer rate in the selective reduction of O2 to water [11,12]. Moreover, exploiting the structural similarities in the active site of Mb and CcO by point mutation, a bio-synthetic models of CcO were created within a Mb scaffold [13]. Heterogeneous and homogeneous oxygen reduction reactions (ORR) using these models reveal the role of the distal metal binding site and the distal proton channel in the rate and selectivity of 4e- / 4H+ reduction from O2 to water [14-17]. Understanding of the role of the proton transfer channel in CcO during mitochondrial respiration was further improved with synthetic Fe-porphyrin active sites with pendant basic groups in the distal pocket. These groups remain protonated at neutral pH, allowing them to functionally mimic the proton transfer residues in the enzyme active site [18]. Despite extensive research, the development of functional models of the Cyt P450 active site has been difficult using conventional synthesis techniques. This is primarily due to the complications associated with the synthesis of stable heme complexes with an axial thiolate ligand [19]. Recently, however, a thiolate-linked Fe porphyrin on a modified Au electrode that can functionally mimic the active site of Cyt P450 has been reported [20,21]. This also helps us to understand the effects of electron transfer rate in catalytic C-H bond activation during enzymatic cycle. In addition, several other functional mimics of heme enzymes have been reported that are beyond the scope of this review.
Employing Heme Enzymes for Novel Chemical Transformations
Understanding the structural and functional relationships of heme enzymes has motivated scientists to expand their catalytic repertoire to achieve new chemical transformations. Through directed evolution and site-directed mutagenesis, heme proteins such as Cyt P450, Mb, and Cyt c have been modified to be excellent catalysts for various reactions such as cyclopropanations, olefinations, N-H, Si-H, and B-H insertion reactions, etc [22-25]. Further reports have shown that replacement of native heme cofactor with Ir porphyrin has also led to enantioselective and siteselective functionalization of C-H and C=C bonds [26]. Active site engineering reached another peak when the peroxidase active site was designed for sulfite reduction by incorporating an Fe4S4 cluster into the active site [27]. This field is still being explored and new results are getting published on a regular basis.
Conclusion
Tremendous progress has been made in elucidating the structure-function relationship in heme proteins and how the protein itself can tune reactivity. The synthetic models have also revealed the minimum features required to mimic an active site of a protein, usually either structurally or functionally. This information was further used to not only mimic enzymatic activity for largescale chemical synthesis, but also to extend catalytic activity for novel chemical transformations.
References
- Banci L, Bertini I (2013) Metallomics and the Cell: Some Definitions and General Comments. Met Ions Life Sci 12: 1-13.
- Poulos T L (2014) Heme Enzyme Structure and Function. Chem Rev 114(7): 3919-3962.
- Bertini I, Cavallaro G, Rosato A (2006) Cytochrome c: Occurrence and Functions. Chem Rev 106(1): 90-115.
- Wikström M, Krab K, Sharma V (2018) Oxygen Activation and Energy Conservation by Cytochrome c Oxidase. Chem Rev 118(5): 2469-2490.
- Sono M, Roach MP, Coulter ED, Dawson JH (1996) Heme-Containing Oxygenases. Chem Rev 96(7): 2841-2888.
- Li H, Poulos TL (1994) Structural variation in heme enzymes: a comparative analysis of peroxidase and P450 crystal structures. Structure 2(6): 461-464.
- Huang X, Groves JT (2018) Oxygen Activation and Radical Transformations in Heme Proteins and Metalloporphyrins. Chem Rev 118(5): 2491-2553.
- Rydberg P, Sigfridsson E, Ryde U (2004) On the role of the axial ligand in heme proteins: a theoretical study. J Biol Inorg Chem 9(2): 203-223.
- Mukherjee M, Dey A (2021) Rejigging Electron and Proton Transfer to Transition between Dioxygenase, Monooxygenase, Peroxygenase, and Oxygen Reduction Activity: Insights from Bioinspired Constructs of Heme Enzymes. JACS Au 1(9): 1296-1311.
- Collman JP, Gagne RR, Reed C, Halbert TR, Lang G, et al. (1975) Picket fence porphyrins. Synthetic models for oxygen binding hemoproteins. J Am Chem Soc 97(6): 1427-1439.
- Collman James P, Devaraj Neal K, Decréau Richard A, Yang Y, Yan YL, et al. (2007) Chidsey Christopher, E. D. A Cytochrome c Oxidase Model Catalyzes Oxygen to Water Reduction Under Rate-Limiting Electron Flux. Science 315(5818): 1565-1568.
- Boulatov R, Collman JP, Shiryaeva IM, Sunderland CJ (2002) Functional Analogues of the Dioxygen Reduction Site in Cytochrome Oxidase: Mechanistic Aspects and Possible Effects of CuB. J Am Chem Soc 124(40): 11923-11935.
- Miner KD, Mukherjee A, Gao YG, Null EL, Petrik ID, et al. (2012) A Designed Functional Metalloenzyme that Reduces O2 to H2O with Over One Thousand Turnovers. Angew Chem Int Ed 51(23): 5589-5592.
- Yu Y, Cui C, Liu X, Petrik ID, Wang J, et al. (2015) A Designed Metalloenzyme Achieving the Catalytic Rate of a Native Enzyme. J Am Chem Soc 137(36): 11570-11573.
- Petrik ID, Davydov R, Kahle M, Sandoval B, Dwaraknath S, et al. (2021) An Engineered Glutamate in Biosynthetic Models of Heme-Copper Oxidases Drives Complete Product Selectivity by Tuning the Hydrogen-Bonding Network. Biochem 60(4): 346-355.
- Mukherjee S, Mukherjee A, Bhagi Damodaran A, Mukherjee M, Lu Y, et al. (2015) A biosynthetic model of cytochrome c oxidase as an electrocatalyst for oxygen reduction. Nat Commun 6: 8467.
- Mukherjee S, Mukherjee M, Mukherjee A, Bhagi Damodaran A, Lu Y, et al. (2018) O2 Reduction by Biosynthetic Models of Cytochrome c Oxidase: Insights into Role of Proton Transfer Residues from Perturbed Active Sites Models of CcO. ACS Catal 8(9): 8915-8924.
- Ghatak A, Bhunia S, Dey A (2020) Effect of Pendant Distal Residues on the Rate and Selectivity of Electrochemical Oxygen Reduction Reaction Catalyzed by Iron Porphyrin Complexes. ACS Catal 10(21): 13136-13148.
- Higuchi T, Uzu S, Hirobe M (1990) Synthesis of a highly stable iron porphyrin coordinated by alkylthiolate anion as a model for cytochrome P-450 and its catalytic activity in oxygen-oxygen bond cleavage. J Am Chem Soc 112(9): 7051-7053.
- Sengupta K, Chatterjee S, Samanta S, Bandyopadhyay S, Dey A (2013) Resonance Raman and Electrocatalytic Behavior of Thiolate and Imidazole Bound Iron Porphyrin Complexes on Self Assembled Monolayers: Functional Modeling of Cytochrome P450. Inorg Chem 52(4): 2000-2014.
- Mukherjee M, Dey A (2019) Electron Transfer Control of Reductase versus Monooxygenase: Catalytic C-H Bond Hydroxylation and Alkene Epoxidation by Molecular Oxygen. ACS Cent Sci 5(4): 671-682.
- Brandenberg OF, Fasan R, Arnold FH (2017) Exploiting and engineering hemoproteins for abiological carbene and nitrene transfer reactions. Curr Opin Biotechnol 47: 102-111.
- Coelho Pedro S, Brustad Eric M, Kannan A, Arnold Frances H (2013) Olefin Cyclopropanation via Carbene Transfer Catalyzed by Engineered Cytochrome P450 Enzymes. Science 339(6117): 307-310.
- Prier CK, Arnold FH (2015) Chemomimetic Biocatalysis: Exploiting the Synthetic Potential of Cofactor-Dependent Enzymes To Create New Catalysts. J Am Chem Soc 137(44): 13992-14006.
- Tinoco A, Steck V, Tyagi V, Fasan R (2017) Highly Diastereo - and Enantioselective Synthesis of Trifluoromethyl-Substituted Cyclopropanes via Myoglobin-Catalyzed Transfer of Trifluoromethylcarbene. J Am Chem Soc 139(15): 5293-5296.
- Oeschger RJ, Larsen MA, Bismuto A, Hartwig JF (2019) Origin of the Difference in Reactivity between Ir Catalysts for the Borylation of C-H Bonds. J Am Chem Soc 141(4): 16479-16485.
- Mirts Evan N, Petrik Igor D, Hosseinzadeh P, Nilges Mark J, Lu Y (2018) A designed heme-[4Fe-4S] metalloenzyme catalyzes sulfite reduction like the native enzyme. Science 361(6407): 1098-1101.